To much of the world, the growing realization that vaccine-induced immunity for COVID-19 eventually fades has come as a shock. But for those of us who lived through the beginnings of the world’s last great pandemic, HIV/AIDS, the tremors feel oddly familiar. With AIDS, we never succeeded in developing an effective vaccine, but we did find medical solutions to treat the disease and control its spread. Those same solutions are what we should be thinking about today for COVID-19 to fill in the gaps where vaccine immunity falters.
HIV and SARS-CoV-2 are two very different viruses, but they hold remarkable similarities: They’re both novel RNA viruses that crossed from animals into humans. They both gave rise to global pandemics, with HIV killing more than 37 million since the pandemic began and SARS-CoV-2 on its way to killing more than five million in its first two years alone. And perhaps most critically, they both employ similar evolutionary mechanisms that allow the viruses to adapt, even under immune pressure.
HIV’s ability to mutate and adapt has made it difficult for us to develop a vaccine against the disease, but we have found other ways to control and slow its spread, primarily through behavior change and antiviral drugs that can treat someone who is infected and, perhaps more importantly, be taken prophylactically by healthy individuals, preventing them from ever becoming infected or ill, even if exposed to the disease. PEP (post-exposure prophylaxis) and PrEP (pre-exposure prophylaxis) are two critical prevention methods for HIV infection that those of us in the scientific community fought long and hard over decades to develop. Today, our community is working at record pace to develop antivirals for COVID-19 but our focus, in my opinion, is far too narrow given all that we have learned with HIV.
The two COVID-19 antivirals receiving the most attention are a polymerase inhibitor called molnupiravir, developed by Merck and Ridgeback, and a protease inhibitor, PF-07321332, developed by Pfizer. The first HIV antivirals were also directed at these same targets. The first antiviral drug for HIV was a polymerase inhibitor, azidothymidine (AZT). The drug works well to stop HIV replication but when administered to patients as a monotherapy, we found it led to the rapid development of resistance. If the virus acquired one or two minor changes in the polymerase, it could render the drug less effective and that variant of the virus could proliferate quickly. Patients living with HIV who received the drug could develop drug resistance within just a few months. A generation of early AIDS victims were brought back from the edge of death only to die from the virus that was AZT resistant.
To overcome challenges of resistance, we broadened the scope to include drugs focused on other targets, like the protease. The idea was to combine drugs that attacked different targets, minimizing the probability of the virus acquiring mutations that would make it resistant to all drugs simultaneously—if one treatment fails to work, other drugs in the cocktail continue the job. The first protease inhibitors against HIV prevented the cleavage of viral polyproteins, which are necessary for both viral replication and the release of mature viral particles from an infected cell. We found that when these drugs were combined with polymerase inhibitors, we could create a combination therapy that was more effective for a longer period of time than protease inhibitors alone.
The combination therapy had another beneficial effect that was important for our success, it widened the drug therapy’s therapeutic index. All drugs have a therapeutic index, which is the ratio between the amount of a drug needed for it to be effective and the amount at which the drug becomes toxic. The index even applies to something as simple as water—the right amount of water keeps you alive, but too much of it and you drown. Patients living with HIV would receive antiviral treatments over their lifetime, so it was important that the drugs could be well tolerated not just over the short term but over an extended period of time. With combination therapies, you could use lower concentrations of each of the drugs with greater effect, which meant that people could survive longer on the treatment without severe side effects.
The therapeutic index is also critical for pre-exposure prophylaxis. With PrEP, we are giving drugs to healthy people so those drugs need to have a very low toxicity. With the early HIV combination therapies, the therapeutic index was tolerable for those infected but the risk of side effects and toxicity was nowhere near where it needed to be to be used prophylactically. We knew prophylactic antivirals was an approach that could work to prevent infection, but we wouldn’t be able to deploy it without finding new drugs with a much wider therapeutic index that could be well tolerated by those infected and those who were healthy, but at risk of exposure.
That set us off on a thirty-year search for better HIV drugs. Taking lessons from other diseases, like cancer, we began expanding our search beyond the polymerase and protease alone, targeting biochemical processes which are unique to the virus and dissimilar to our biochemistry. Our bodies make and use polymerases and proteases regularly for day-to-day minute-by-minute functions. While a virus’ protease may not be exactly like our own cell’s protease, it may be similar enough that protease inhibitors developed to target a virus may take a toll on a patient’s healthy cells as well.
In the end, that hunt was successful. My own work looking beyond the prime protease and polymerase targets led to two additional targets that have proven well worth the time and effort to explore them: the integrase and capsid. Indeed, these two targets are at the root of two new long-acting HIV drugs that have a much higher therapeutic index than any previous. Lenacapivir, a long-acting drug from Gilead, is a capsid inhibitor that works on the integration pathway. By binding to the capsid protein, the drug blocks HIV’s ability to interact with a cellular chaperone that would normally allow it entry into a cell’s nucleus. Stop the virus from entering the nucleus and you stop it from replicating. Cabotegravir, developed by ViiV Healthcare, also works on the integration complex, this time focusing instead on a viral enzyme critical to integration: the integrase. An integrase strand inhibitor, it blocks the virus from integration, blocking its ability to replicate and then spread. We also now have a wealth of prime targets for HIV drugs, beyond the integrase and capsid, including anti-Tat protein, anti-Rev protein, and anti-endonuclease inhibitors.
Our success with HIV, after decades of trial and error, should now be applied to COVID-19. If we want drugs with a high therapeutic index that we can use prophylactically over the long term, we need to look beyond the polymerase and protease and start thinking about combination therapies. The two COVID-19 antivirals farthest along in development today are, as I mentioned at the beginning of this column, a polymerase inhibitor called molnupiravir and a protease inhibitor, PF-07321332.
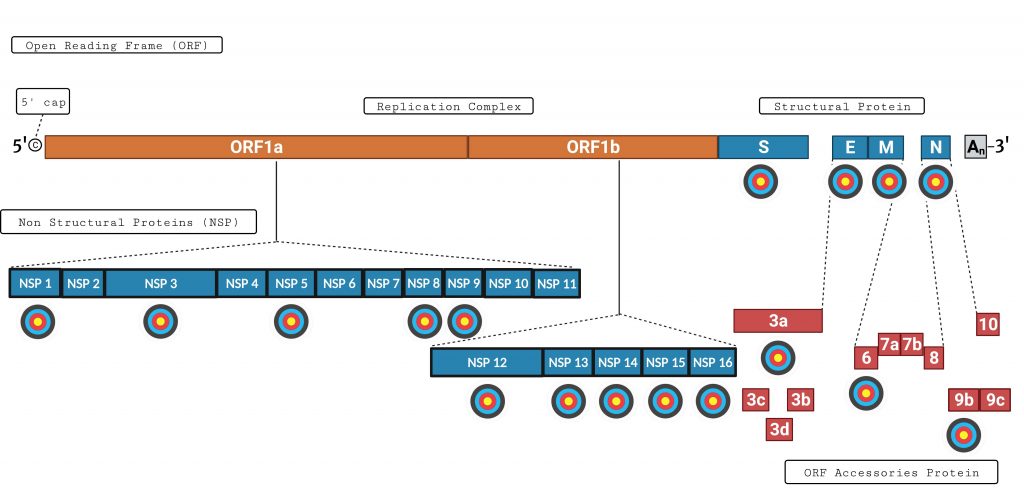
Molnupiravir works by attacking SARS-CoV-2’s polymerase, the enzyme that builds the viral genome during replication. The drug tricks the virus into using molnupiravir for replication, then inserts errors into the virus’ genetic code once replication is underway. The drug is a tautomer that assumes two forms, one which closely resembles uracil (U) and the other cytosine (C). Once it is recopied, the replicating polymerase develops transition mutations, where a U nucleotide is converted to a C and a C to U. When enough copying errors occur, the virus is essentially killed off, unable to replicate any further which shortens the length of a person’s infection, and limits onward transmission. If administered prophylactically, it may prevent an infection from ever taking hold.
Molnupiravir comes with some important caveats. First, is the potential for resistance, if administered as a monotherapy. This, as we’ve discussed, is common to all antivirals and can be solved through combination therapy. Second though, is its potential to supercharge the creation of SARS-CoV-2 variants if administered in suboptimal concentrations. If rolled out globally, the possibility that not all individuals will take the full five-day course of treatments is very real. It’s possible that at these suboptimal concentrations, the drug may introduce mutations in SARS-CoV-2 that drastically change how the virus operates, but doesn’t necessarily stop it from replicating. That could lead to new and potentially more virulent variants. Third, and perhaps most importantly, is its potential mutagenicity in human beings. A study published earlier this year finds that, once inside a cell, the active form of molnupiravir may be both genotoxic and mutagenic to our own healthy cells, potentially leading to the growth of cancerous tumors or birth defects, either in a developing fetus or by being incorporated into sperm precursor cells.
We know less about the potential red flags with Pfizer’s drug candidate, which targets the protease instead of the polymerase, blocking the activity of the main enzyme that SARS-CoV-2 needs to replicate and preventing the virus from cleaving long protein chains into the parts it needs to reproduce itself. But we know, at the very least, that it will run into the same challenge as all antivirals if administered as a monotherapy, which is resistance. Focusing COVID antiviral drug development solely on the protease and polymerase is short-sighted at best and likely to lead to new variants that are more resistant to these drugs’ effects.
The good news is that SARS-CoV-2 offers us a wealth of other exciting drug targets, just like HIV. The image of the SARS-CoV-2 genome below shows some of the targets that should be considered for antiviral drug development.
NSP 1, for example, is the first protein made and performs critical functions in virus replication by interacting with a very specific RNA, making it a very desirable target. NSP 3 has at least seven different enzymatic functions and inhibition of any one of those functions should inhibit virus replication. It is a target-rich environment, including an ADP-ribosylation site and a papein protease site. Below is a chart that highlights some of the key proteins, their function, and critical antiviral drug development strategies (see page 48).
Our years of research and exploration on other infectious diseases like HIV have taught us what works and what doesn’t when it comes to antivirals. With influenza, we have done what we should be doing for COVID-19: deploying antivirals in combination with vaccines to create multiple rings of protection around those at risk of infection. The newer oral antivirals for flu, like baloxavir marboxil, can significantly reduce transmission of influenza among people living in the same house, meaning that even if one person in a household or communal living space falls ill, they can continue living in the same environment without necessarily infecting those around them.
This should be the same focus for COVID drug makers moving forward: continue to improve the quality and durability of our vaccines while developing combinations of antiviral drugs focused on a much wider array of targets, thus ensuring their safety and sustainability over the long term. This can’t be done in a vacuum, however. The efforts of the global research community—universities, research institutes, and biotechnology and pharmaceutical companies—should now expand to include a heavy focus on antiviral drug development, something that can only be done with focused funding and government leadership. The magic drug combination may come from the work of multiple labs, not one single company, and the government is the natural entity to accelerate research and coordinate clinical trials and testing of potential drugs.
This was the approach that eventually led to our success against HIV and has become routine for other diseases like cancer. If we are successful with COVID-19, we will not only have created another powerful tool to control this pandemic—alongside vaccine and public health control measures—but we will have laid the groundwork for a more rapid response against future emerging infectious diseases.
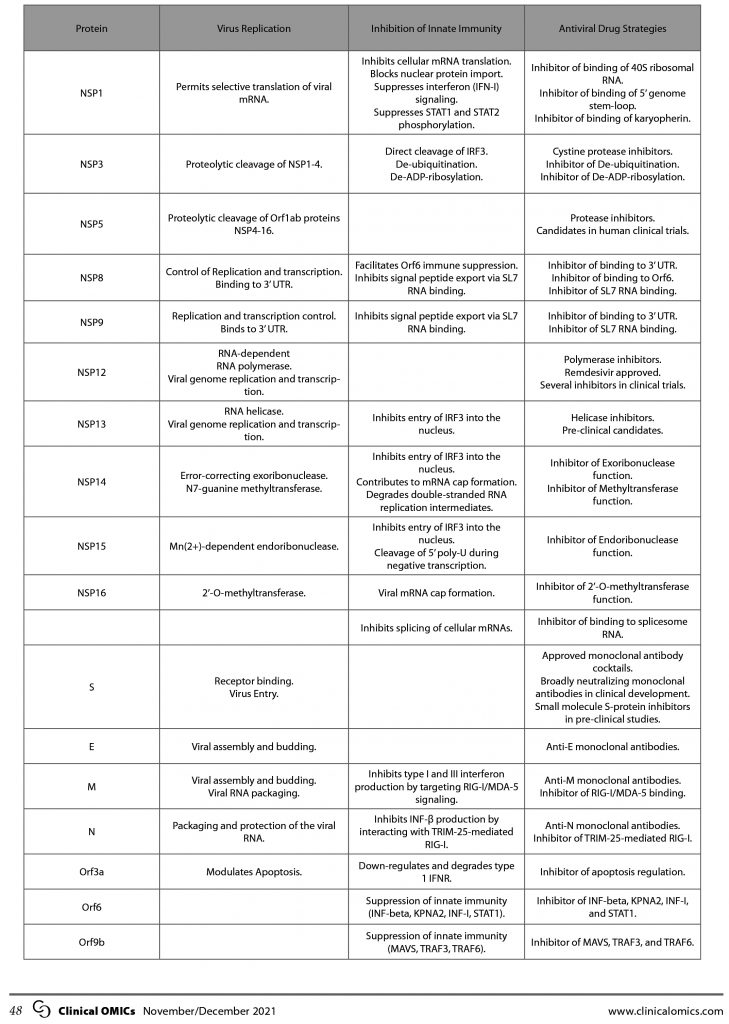